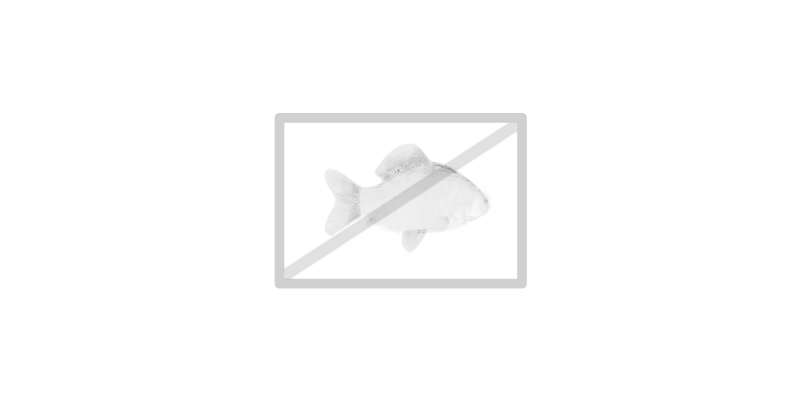
Information
Author: Caroline Marques Maia
Version: C | 1.0Published: 2025-03-27
WelfareScore | farm
The score card gives our welfare assessments for aquatic species in 10 criteria.
For each criterion, we score the probability to experience good welfare under minimal farming conditions ("Likelihood") and under high-standard farming conditions ("Potential") representing the worst and best case scenario. The third dimension scores how certain we are of our assessments based on the number and quality of sources we found ("Certainty").
The WelfareScore sums just the "High" scores in each dimension. Although good welfare ("High") seems not possible in some criteria, there could be at least a potential improvement from low to medium welfare (indicated by ➚ and the number of criteria).
- Li = Likelihood that the individuals of the species experience good welfare under minimal farming conditions
- Po = Potential of the individuals of the species to experience good welfare under high-standard farming conditions
➚ = potential improvements not reaching "High" - Ce = Certainty of our findings in Likelihood and Potential
WelfareScore = Sum of criteria scoring "High" (max. 10 per dimension)
General remarks
Israeli fish farmers started using an Oreochromis niloticus ♀ x O. aureus ♂ hybrid in the 1960s aiming to prevent uncontrolled propagation of tilapias in production ponds. It is also cultured in Saudi Arabia, with a great interest for aquaculture, especially because it has a better growth and yield than O. niloticus, O. aureus, and O. mossambicus. Furthermore, among several interspecific tilapia hybrids, O. niloticus x O. aureus has shown to be the most suitable one in terms of growth rate, sex ratio, cold tolerance, and body coloration. Stocking hormonally sex reversed FINGERLINGS has become the generalised practice to cope with the uncontrolled spawning issue under farming conditions. Usually, only males are farmed, but 2-3% of the sex-reversed FINGERLINGS remain as females which are still able to spawn in grow-out ponds. Thus, predator species are used to feed on these unwanted eggs, LARVAE, and FRY in warm freshwater aquaculture ponds.
Currently, important information about this hybrid is still missing in the literature, making it difficult to better assess its welfare in farms. Considering wild information, findings about home range as well as aggression and migratory distances is still missing for the parental species O. niloticus, whereas there are important knowledge gaps about home range, depth range, and migration patterns for the parental species O. aureus. Further research on reproduction, stress response, and malformations in farms are urgently needed. Moreover, important farming information about aggregation for the early life stages and substrate use for these age classes and SPAWNERS is still missing.
Note: because this is a hybrid species, we refer to the wild needs and behaviours of the parent species, Oreochromis niloticus and O. aureus, where available, in order to compare them with what the hybrid is provided with in captivity. For a hybrid species, however, welfare studies on the current farming conditions might be even more important than these wild needs.
1 Home range
Many species traverse in a limited horizontal space (even if just for a certain period of time per year); the home range may be described as a species' understanding of its environment (i.e., its cognitive map) for the most important resources it needs access to.
What is the probability of providing the species' whole home range in captivity?
It is unclear for minimal and high-standard farming conditions given we did not find wild data for JUVENILES and ADULTS of the parent species O. niloticus and O. aureus. Our conclusion is based on a medium amount of evidence, as further research is needed on home range in the wild but – maybe more importantly in a hybrid species – on welfare under current farming conditions.


2 Depth range
Given the availability of resources (food, shelter) or the need to avoid predators, species spend their time within a certain depth range.
What is the probability of providing the species' whole depth range in captivity?
It is low for minimal farming conditions given up to 4-30 m depth range in O. niloticus and in case the unclear depth range is fully used (mean 9 m) in O. aureus, as ponds, cages, and tanks do not cover the whole range in the wild. It is medium for high-standard farming conditions, as the mentioned systems at least overlap with the range in the wild. Our conclusion is based on a medium amount of evidence, as further research is needed on depth range in the wild and – maybe more importantly in a hybrid species – on welfare under current farming conditions.


3 Migration
Some species undergo seasonal changes of environments for different purposes (feeding, spawning, etc.), and to move there, they migrate for more or less extensive distances.
What is the probability of providing farming conditions that are compatible with the migrating or habitat-changing behaviour of the species?
It is low for minimal farming conditions given that the parent species O. niloticus and O. aureus undertake more or less extensive migrations (even though of partly unknown distance), and we cannot be sure that providing each age class with their respective environmental conditions will satisfy their urge to migrate or whether they need to experience the transition. It is unclear for high-standard farming conditions, as the lower end of the migration distance is unknown and therefore also the degree to which the space range in captivity potentially overlaps with it. Our conclusion is based on a medium amount of evidence, as further research is needed on specific migration distances in the wild and – maybe more importantly in a hybrid species – on welfare under current farming conditions.


4 Reproduction
A species reproduces at a certain age, season, and sex ratio and possibly involving courtship rituals.
What is the probability of the species reproducing naturally in captivity without manipulation of these circumstances?
It is high for minimal and high-standard farming conditions, as natural breeding (without manipulation) with farm-reared IND is possible and verified for the farming context. Our conclusion is based on a medium amount of evidence, as further research is needed on reproduction behaviour in the wild and – maybe more importantly in a hybrid species – on welfare under current farming conditions.


5 Aggregation
Species differ in the way they co-exist with conspecifics or other species from being solitary to aggregating unstructured, casually roaming in shoals or closely coordinating in schools of varying densities.
What is the probability of providing farming conditions that are compatible with the aggregation behaviour of the species?
It is low for minimal farming conditions, as densities in hapas, cages, tanks, and some ponds go beyond the smallest density of the parent species O. niloticus and O. aureus in the wild (although we cannot be sure in all age classes). It is medium for high-standard farming conditions, as densities in other ponds at least overlap with the density range in the wild. Our conclusion is based on a high amount of evidence, unless farm studies show that hybrid tilapia is well under higher densities than its parent species in the wild.


6 Aggression
There is a range of adverse reactions in species, spanning from being relatively indifferent towards others to defending valuable resources (e.g., food, territory, mates) to actively attacking opponents.
What is the probability of the species being non-aggressive and non-territorial in captivity?
It is low for minimal farming conditions, as there are hints for aggression in all age classes. It is medium for high-standard farming conditions, as ways to reduce (but not avoid) aggression (amount feeding, keeping separate, size grading, density) are verified for the farming context. Our conclusion is based on a medium amount of evidence, as further (species-specific) research is needed.


7 Substrate
Depending on where in the water column the species lives, it differs in interacting with or relying on various substrates for feeding or covering purposes (e.g., plants, rocks and stones, sand and mud, turbidity).
What is the probability of providing the species' substrate and shelter needs in captivity?
It is low for minimal farming conditions, as almost all age classes of the parent species O. niloticus and O. aureus use substrate, but hapas, cages, and some ponds and tanks are devoid of it. It is medium for high-standard farming conditions given a) eggs are left in female’s mouth, b) earthen ponds with (plastic) substrate for FRY to ADULTS which are not replaced by tarpaulin or concrete bottom, and given c) natural reproduction with spawning substrate in ponds or cages for SPAWNERS which need to be verified for the hybrid. Our conclusion is based on a medium amount of evidence, as further species-specific research is needed


8 Stress
Farming involves subjecting the species to diverse procedures (e.g., handling, air exposure, short-term confinement, short-term crowding, transport), sudden parameter changes or repeated disturbances (e.g., husbandry, size-grading).
What is the probability of the species not being stressed?
It is low for minimal farming conditions, as the parent species O. niloticus and O. aureus are stressed (water quality, handling, confinement, crowding, transport). It is medium for high-standard farming conditions, as most ways to reduce (but not avoid) stress need to be verified for the hybrid. Our conclusion is based on a low amount of evidence, as further species-specific research is needed.


9 Malformations
Deformities that – in contrast to diseases – are commonly irreversible may indicate sub-optimal rearing conditions (e.g., mechanical stress during hatching and rearing, environmental factors unless mentioned in crit. 3, aquatic pollutants, nutritional deficiencies) or a general incompatibility of the species with being farmed.
What is the probability of the species being malformed rarely?
It is high for minimal and high-standard farming conditions, as malformation rates of the parent species O. niloticus does not exceed 10%. Our conclusion is based on a low amount of evidence, as further species-specific research is needed.


10 Slaughter
The cornerstone for a humane treatment is that slaughter a) immediately follows stunning (i.e., while the individual is unconscious), b) happens according to a clear and reproducible set of instructions verified under farming conditions, and c) avoids pain, suffering, and distress.
What is the probability of the species being slaughtered according to a humane slaughter protocol?
It is low for minimal farming conditions (asphyxia, hypothermia, live exsanguination, live filleting). It is medium for high-standard farming conditions, as electrical stunning, followed by exsanguination, evisceration, or filleting, induces unconsciousness fast (if done correctly), kills while still unconscious, but needs to be verified for the hybrid. Our conclusion is based on a low amount of evidence, as further species-specific research is needed.


Side note: Domestication
Teletchea and Fontaine introduced 5 domestication levels illustrating how far species are from having their life cycle closed in captivity without wild input, how long they have been reared in captivity, and whether breeding programmes are in place.
What is the species’ domestication level?
Hybrid: DOMESTICATION LEVEL 5 18, fully domesticated.
Side note: Forage fish in the feed
450-1,000 milliard wild-caught fishes end up being processed into fish meal and fish oil each year which contributes to overfishing and represents enormous suffering. There is a broad range of feeding types within species reared in captivity.
To what degree may fish meal and fish oil based on forage fish be replaced by non-forage fishery components (e.g., poultry blood meal) or sustainable sources (e.g., soybean cake)?
All age classes:
- WILD: O. niloticus: opportunistic – either mainly herbivorous 7 (non-native habitat: 44 43 48 123 82) or mainly omnivorous (non-native habitat: 4 47 42 49). O. aureus: native (artificial lake) and non-native habitat: omnivorous 124 54 125 81, but mainly phytoplankton 10, plants and algae 54. FRY: feed on zooplankton 63.
- FARM: ponds: hard plastic substrates for periphyton growth equivalent to 50% of the pond surface may reduce up to 40% in the feed amount supplied 30. Fish meal may be completely* replaced by non-forage fishery components, but with the inclusion of fish oil 26.
- LAB: JUVENILES: fish meal may be completely* replaced by sustainable sources in fresh water but not in brackish water (20%) – with even higher mortality 126; fish oil may be mostly* and fish meal may be completely* replaced by a mix of sustainable sources and non-forage fishery components 127; fish meal may be partly* 128 129 or mostly* 129 replaced by sustainable sources but with an increase in fish oil; fish meal may be partly* replaced by sustainable sources 130; fish meal may be completely* replaced by non-forage fishery components 131; fish oil may be completely* replaced by sustainable sources 132. JUVENILES-ADULTS: fish meal and fish oil may be partly* replaced by sustainable sources 133.
*partly = <51% – mostly = 51-99% – completely = 100%
Side note: Commercial relevance
How much is this species farmed annually?
410,553 t/year 1990-2019 amounting to estimated 681,000,000 IND/year 1990-2019 134.
Glossary
BENTHOPELAGIC = living and feeding near the bottom of a body of water, floating above the floor
BIOFLOC = dense microbial communities growing in flocs 35
DOMESTICATION LEVEL 5 = selective breeding programmes are used focusing on specific goals 122
FARM = setting in farming environment or under conditions simulating farming environment in terms of size of facility or number of individuals
FINGERLINGS = early juveniles with fully developed scales and working fins, the size of a human finger
FRY = larvae from external feeding on
IND = individuals
JUVENILES = fully developed but immature individuals
LAB = setting in laboratory environment
LARVAE = hatching to mouth opening
MOUTHBROODER = also "mouthbreeder"; parent taking eggs into the mouth after fertilisation
NTU = Nephelometric Turbidity Units
PHOTOPERIOD = duration of daylight
POTAMODROMOUS = migrating within fresh water
SPAWNERS = adults during the spawning season; in farms: adults that are kept as broodstock
TOTAL LENGTH = from snout to tip of caudal fin as compared to fork length (which measures from snout to fork of caudal fin) or standard length (from head to base of tail fin) or body length (from the base of the eye notch to the posterior end of the telson) 34
WILD = setting in the wild
Bibliography
2 Komolafe, O. O., and G. a. O. Arawomo. 2007. Reproductive strategy of Oreochromis niloticus (Pisces: Cichlidae) in Opa reservoir, Ile-Ife, Nigeria. Revista de Biología Tropical 55: 595–602.
3 Lowe-McConnell, Rosemary H. 1959. Breeding behaviour patterns and ecological differences between tilapia species and their significance for evolution within the genus tilapia (Pisces: Cichlidae). Proceedings of the Zoological Society of London 132: 1–30. https://doi.org/10.1111/j.1469-7998.1959.tb05510.x.
4 Balirwa, J. S. 1998. Lake Victoria wetlands and the ecology of the Nile tilapia, Oreochromis niloticus Linne. Ph.D. dissertation, Wageningen, The Netherlands: Wageningen Agricultural University.
5 Peterson, Mark S., William T. Slack, Nancy J. Brown-Peterson, Jennifer L. McDonald, and C. M. Taylor. 2004. Reproduction in Nonnative Environments: Establishment of Nile Tilapia, Oreochromis niloticus, in Coastal Mississippi Watersheds. Copeia 2004: 842–849. https://doi.org/10.1643/CE-04-134R1.
6 Lowe (McConnell), Rosemary H. 1955. The Feduncity of Tilapia Species. The East African Agricultural Journal 21: 45–52.
7 Hopson, A. J., A. A. Q. R McLeod, B. J. Harbott, and J. T. N. Ogari. 1982. The biology of perciform fishes. In A report on the findings of the Lake Turkana project 1972-1975, ed. A. J. Hopson, 5:1283–1502. London: Overseas Development Administration.
8 Boulenger, Charles L. 1908. On the Breeding‐Habits of a Cichlid Fish ( Tilapia nilotica). Proceedings of the Zoological Society of London 78: 405–407. https://doi.org/10.1111/j.1096-3642.1908.tb01851.x.
9 Liebman, E. 1933. Some Observations on the Breeding Habits of Palestine Cichlidæ. Proceedings of the Zoological Society of London 103: 885–888. https://doi.org/10.1111/j.1096-3642.1933.tb01632.x.
10 Ben-Tuvia, A. 1959. The biology of the cichlid fishes of Lakes Tiberias and Huleh. Bulletin of the Research Council of Israel B Zoology 8B: 153–188.
11 Payne, A. I., and R. I. Collinson. 1983. A comparison of the biological characteristics of Sarotherodon niloticus (L.) with those of S. aureus (Steindachner) and other tilapia of the delta and lower Nile. Aquaculture 30: 335–351. https://doi.org/10.1016/0044-8486(83)90174-6.
12 Behrends, L. L., J. B. Kingsley, and A. H. Price III. 1993. Hatchery production of blue tilapia, Oreochromis aureus (Steindachner), in small suspended hapa nets. Aquaculture Research 24: 237–243. https://doi.org/10.1111/j.1365-2109.1993.tb00546.x.
13 Ridha, M. T. 2004. Observations on the reproductive performance of three mouth-brooding tilapia species in low-salinity underground water. Aquaculture Research 35: 1031–1038. https://doi.org/10.1111/j.1365-2109.2004.01110.x.
14 Stickney, R.R. 2017. Tilapia Feeding Habits and Environmental Tolerances. In Tilapia in Intensive Co-culture, 25–35. John Wiley & Sons, Ltd. https://doi.org/10.1002/9781118970652.ch2.
15 Al-Wan, Sara M., and Abdul-Razak M. Mohamed. 2019. Analysis of the Biological Features of the Blue Tilapia, Oreochromis aureus in the Garmat Ali River, Basrah, Iraq. Asian Journal of Applied Sciences 07: 776–787.
16 McBay, L. G. 1961. The biology of Tilapia nilotica. In Proc. 15th ann. Conf. Southeastern Ass. Game Fish Commissioners, 208–218.
17 Trewavas, Ethelwynn. 1983. Tilapiine Fishes of the Genera Sarotherodon, Oreochromis and Danakilia. [Published in cooperation with] British Museum (Natural History) [by] Comstock Pub. Associates, a division of Cornell University Press.
18 Maia, Caroline Marques. 2024. Conclusion.
19 Hulata, G., G. W. Wohlfarth, I. Karplus, G. L. Schroeder, S. Harpaz, A. Halevy, S. Rothbard, S. Cohen, I. Israel, and M. Kavessa. 1993. Evaluation of Oreochromis niloticus×O. aureus hybrid progeny of different geographical isolates, reared under varying management regimes. Aquaculture 115: 253–271. https://doi.org/10.1016/0044-8486(93)90141-K.
20 Siddiqui, A.Q., and A.H. Al-Harbi. 1997. Effects of sex ratio, stocking density and age of hybrid tilapia on seed production in concrete tanks in Saudi Arabia. Aquaculture International 5: 207–216. https://doi.org/10.1023/A:1018383201054.
21 Siddiqui, A Q, A H Al-Harbi, and Y S Al-Hafedh. 1997. Effects of food supply on size at first maturity, fecundity and growth of hybrid tilapia, Oreochromis niloticus (L.) × Oreochromis aureus (Steindachner), in outdoor concrete tanks in Saudi Arabia. Aquaculture Research 28: 341–349. https://doi.org/10.1046/j.1365-2109.1997.t01-1-00864.x.
22 Milstein, A., Y. Eran, E. Nitzan, M. Zoran, and D. Joseph. 2000. Tilapia wild spawning control through predator fishes: Israelitrial with red-drum and hybrid bass. Aquaculture International 8: 31–40. https://doi.org/10.1023/A:1009268815430.
23 Milstein, A., D. Joseph, Y. Peretz, and S. Harpaz. 2005. Evaluation of organic tilapia culture in periphyton-based ponds. The Israeli Journal of Aquaculture 57: 143–155.
24 Schlechtriem, C., V. Bresler, L. Fishelson, M. Rosenfeld, and K. Becker. 2004. Protective effects of dietary l-carnitine on tilapia hybrids (Oreochromis niloticus x Oreochromis aureus) reared under intensive pond-culture conditions. Aquaculture Nutrition 10: 55–63. https://doi.org/10.1046/j.1365-2095.2003.00283.x.
25 Crab, R., M. Kochva, W. Verstraete, and Y. Avnimelech. 2009. Bio-flocs technology application in over-wintering of tilapia. Aquacultural Engineering 40: 105–112. https://doi.org/10.1016/j.aquaeng.2008.12.004.
26 Huan, D., X. Li, M. A. K. Chowdhury, H. Yang, G. Liang, and X. Leng. 2018. Organic acid salts, protease and their combination in fish meal-free diets improved growth, nutrient retention and digestibility of tilapia (Oreochromis niloticus × O. aureus). Aquaculture Nutrition 24: 1813–1821. https://doi.org/10.1111/anu.12820.
27 Díkel, S. 1997. Effect of Different Stocking Densities on Growth of Hybrid Tilapia (Oreochromis aureus x O. niloticus) in Cages Standing in Concrete Ponds. Turkish Journal of Veterinary & Animal Sciences 21: 247–250. https://doi.org/-.
28 Doudet, T. 1992. Brackishwater tolerance of some species and hybrids of Oreochromis for use in lagoon aquaculture (Ivory Coast). Aquaculture 102: 275–288. https://doi.org/10.1016/0044-8486(92)90154-D.
29 Ray, C. L., J. W. Abernathy, B. W. Green, A. R. Rivers, K. K. Schrader, S. D. Rawles, M. E. McEntire, M. D. Lange, and C. D. Webster. 2024. Effect of dietary phytase on water and fecal prokaryotic and eukaryotic microbiomes in a hybrid tilapia (Oreochromis aureus x O. niloticus) mixotrophic biofloc production system. Aquaculture 581: 740433. https://doi.org/10.1016/j.aquaculture.2023.740433.
30 Milstein, A., Y. Peretz, and S. Harpaz. 2008. Culture of organic tilapia to market size in periphyton-based ponds with reduced feed inputs. Aquaculture Research 40: 55–59. https://doi.org/10.1111/j.1365-2109.2008.02062.x.
31 McDonald, Jennifer L., Mark S. Peterson, and William T. Slack. 2007. Morphology, Density, and Spatial Patterning of Reproductive Bowers in an Established Alien Population of Nile Tilapia, Oreochromis niloticus. Journal of Freshwater Ecology 22: 461–468. https://doi.org/10.1080/02705060.2007.9664176.
32 Bandara, K.V. Sandun N., and Upali S. Amarasinghe. 2017. Factors Related to Nesting Sites of Oreochromis niloticus (Linnaeus 1758; Cichlidae) in Irrigation Reservoirs, Sri Lanka. Asian Fisheries Science 30: 319–335. https://doi.org/10.33997/j.afs.2017.30.4.008.
33 Turner, G. F., and R. L. Robinson. 2000. Reproductive biology, mating systems and parental care. In Tilapias: Biology and Exploitation, ed. Malcolm C. M. Beveridge and Brendan J. McAndrew, 33–58. Dordrecht: Springer Netherlands.
34 Pawson, M.G., and G.D. Pickett. 1996. The Annual Pattern of Condition and Maturity in Bass, Dicentrarchus Labrax, in Waters Around England and Wales. Journal of the Marine Biological Association of the United Kingdom 76: 107. https://doi.org/10.1017/S0025315400029040.
35 Schveitzer, Rodrigo, Rafael Arantes, Manecas Francisco Baloi, Patrícia Fóes S. Costódio, Luis Vinatea Arana, Walter Quadros Seiffert, and Edemar Roberto Andreatta. 2013. Use of artificial substrates in the culture of Litopenaeus vannamei (Biofloc System) at different stocking densities: Effects on microbial activity, water quality and production rates. Aquacultural Engineering 54: 93–103. https://doi.org/10.1016/j.aquaeng.2012.12.003.
36 Hopson, A. J., B. J. Harbott, and A. A. Q. R McLeod. 1982. Shore survey. In A report on the findings of the Lake Turkana project 1972-1975, ed. A. J. Hopson, 2:557–580. London: Overseas Development Administration.
37 Fishelson, L. 1967. Cichlidae of the genus Tilapia in Israel. Bamidgeh 18: 67–80.
38 Kodukhova, Yu. V., and D. P. Karabanov. 2023. Finding of the Blue Tilapia Oreochromis aureus (Cichlidae) in the Gorky Reservoir (Volga River). Inland Water Biology 16: 577–582. https://doi.org/10.1134/S1995082923030112.
39 Hopson, A. J., and J. Hopson. 1982. The fishes of Lake Turkana. In A report on the findings of the Lake Turkana project 1972-1975, ed. A. J. Hopson, 1:281–348. London: Overseas Development Administration.
40 Hopson, A. J., J. Hopson, A. A. Q. R McLeod, and M. G. Pawson. 1982. Openwater surveys. In A report on the findings of the Lake Turkana project 1972-1975, ed. A. J. Hopson, 2:581–750. London: Overseas Development Administration.
41 Balirwa, J. S. 1990. The present status of the fishery for tilapia in Lake Victoria (Uganda). In , 61–64.
42 Njiru, M., J. E. Ojuok, J. B. Okeyo-Owuor, M. Muchiri, M. J. Ntiba, and I. G. Cowx. 2006. Some biological aspects and life history strategies of Nile tilapia Oreochromis niloticus (L.) in Lake Victoria, Kenya: Biology of Nile tilapia in Lake Victoria. African Journal of Ecology 44: 30–37. https://doi.org/10.1111/j.1365-2028.2006.00610.x.
43 Goudswaard, K., J.H. Wanink, F. Witte, E.F.B. Katunzi, M.R. Berger, and D.J. Postma. 2004. Diel vertical migration of major fish-species in Lake Victoria, East Africa. Hydrobiologia 513: 141–152. https://doi.org/10.1023/B:hydr.0000018179.80116.93.
44 Bwanika, G. N., B. Makanga, Y. Kizito, L. J. Chapman, and J. Balirwa. 2004. Observations on the biology of Nile tilapia, Oreochromis niloticus L., in two Ugandan crater lakes. African Journal of Ecology 42: 93–101. https://doi.org/10.1111/j.1365-2028.2004.00468.x.
45 van Oijen, M. J. P. 1995. Appendix I. Key to Lake Victoria fishes other than haplochromine cichlids. In Fish Stocks and Fisheries of Lake Victoria: A Handbook for Field Observations, ed. Frans Witte and Wim L. T. van Densen, 209–300. Dyfed, Great Britain: Samara Publishing Limited.
46 Froese, R., and D. Pauly. 2014. FishBase. World Wide Web electronic publication. www.fishbase.org.
47 Bwanika, G. N., L. J. Chapman, Y. Kizito, and J. Balirwa. 2006. Cascading effects of introduced Nile perch (Lates niloticus) on the foraging ecology of Nile tilapia (Oreochromis niloticus). Ecology of Freshwater Fish 15: 470–481. https://doi.org/10.1111/j.1600-0633.2006.00185.x.
48 Oso, J. A., I. A. Ayodele, and O. Fagbuaro. 2006. Food and Feeding Habits of Oreochromis niloticus (L.) and Sarotherodon galilaeus (L.) in a Tropical Reservoir. World Journal of Zoology 1: 118–121.
49 Peterson, Mark S., William T. Slack, Gretchen L. Waggy, Jeremy Finley, Christa M. Woodley, and Melissa L. Partyka. 2006. Foraging in Non-Native Environments: Comparison of Nile Tilapia and Three Co-Occurring Native Centrarchids in Invaded Coastal Mississippi Watersheds. Environmental Biology of Fishes 76: 283–301. https://doi.org/10.1007/s10641-006-9033-4.
50 Froese, R., and D. Pauly. 2024. Oreochromis aureus, Blue tilapia: fisheries, aquaculture. World Wide Web electronic publication. FishBase.
51 Cummings, D., M. Goren, A. Gasith, and T. Zohary. 2017. Inundated shore vegetation as habitat for cichlids breeding in a lake subjected to extreme water level fluctuations. Inland Waters 7: 449–460. https://doi.org/10.1080/20442041.2017.1388984.
52 Saeed, Samir M. 2013. Impact of environmental parameters on fish condition and quality in Lake Edku, Egypt. Egyptian Journal of Aquatic Biology and Fisheries 17: 101–112.
53 Abdel Aziz, N. E., and S. M. Aboul Ezz. 2004. The structure of zooplankton community in lake Maryout, Alexandria, Egypt. Egyptian Journal of Aquatic Research 30: 160–170.
54 Gophen, M., Y. Yehuda, A. Malinkov, and G. Degani. 1998. Food composition of the fish community in Lake Agmon. Hydrobiologia 380: 49–57. https://doi.org/10.1023/A:1003225210226.
55 Bakhoum, Shnoudy A., and M. Abdallah. 2002. Study to Detect Impacts of Pollution on Fishery Biology of Tilapias in Lake Manzalah, Egypt 13. https://doi.org/10.4197/mar.13-1.8.
56 Mehanna, Sahar F., Ibrahim M. Shaker, and Alam El-Deen Farouk. 2014. Impacts of excessive fishing effort and heavy metals pollution on the tilapia production from Lake Manzalah. In , 57–74.
57 Abdelkarim, Mohamad S., Dalia M. Belal, Nasser S. Flefil, Mahmoud H. Hegab, Abeer M. Mahmoud, Afify D. Al-Afify, Walid Aly, and Mohamed H. Ali. 2023. Ecosystem and Commercializing of Fish in a Rich-Minerals, Low-Salinity Closed Lake. Water, Air, & Soil Pollution 234: 626. https://doi.org/10.1007/s11270-023-06637-6.
58 Jawad, Laith A., Fawziah Sh. Habbeb, and Mustafa A. Al-Mukhtar. 2018. Morphometric and Meristic Characters of Two Cichlids, Coptodon zillii and Oreochromis aureus Collected from Shatt al-Arab River, Basrah, Iraq. International Journal of Marine Science 8: 12–24.
59 Abd El-Satar, Amaal, and A.A. Elewa. 2001. Water Quality and Environmental Assessments of the River Nile at Rosetta branch. In Proceedings of the 2nd International Conference and Exhibition for Life and Environment, 136–164. Alexandria, Egypt.
60 Mahmoud, H. Hatem, and M. Marwa Mazrouh. 2008. Biology and fisheries management of tilapia species in Rosetta branch of the Nile river, Egypt. Egyptian Journal of Aquatic Research 34: 272–284.
61 Riede, K. 2004. Global register of migratory species - from global to regional scales. Final report of the R&D Projekt 808 05 081. Bonn, Germany: Federal Agency for Nature Conservation.
62 Chervinski, Jonathan. 1968. The cichlids of Ein-Feshkha springs I. Tilapia aurea exul (Steinitz). Hydrobiologia 32: 150–156. https://doi.org/10.1007/BF00179545.
63 Alzeny, A., N. E. Abdel-Aziz, A. E. El-Ghobashy, and W. S. El-Tohamy. 2024. Diet Composition and Feeding Habits of Fish Larvae of Five Species in the Burullus Lake, Egypt. Thalassas: An International Journal of Marine Sciences. https://doi.org/10.1007/s41208-024-00677-3.
64 Lung’ayia, H. B. O. 1994. Some aspects of the reproductive biology of the Nile tilapia Oreochromis niloticus (L) in the Nyanza Gulf of Lake Victoria, Kenya. In Proceedings of the Second EEC Regional Seminar on Recent Trends of Research on Lake Victoria Fisheries, ed. E. Okemwa, E. O. Wakwabi, and A. Getabu, 121–127. Nairobi: ICIPE SCIENCE.
65 Ainou, H., J. Panfili, A. Pariselle, M. Labonne, H. Louizi, A. Benhoussa, O.B. Rkhami, and JF Agnèse. 2023. Life-history traits in two invasive species of tilapias in Morocco. African Journal of Aquatic Science 48: 223–235. https://doi.org/10.2989/16085914.2023.2197464.
66 Chervinski, J., and M. Zorn. 1974. Note on the growth of Tilapia aurea (Steindachner) and Tilapia zillii (Gervais) in sea-water ponds. Aquaculture 4: 249–255. https://doi.org/10.1016/0044-8486(74)90037-4.
67 Bakhoum, Shnoudy. 2002. Comparitive reproductive biology of the Nile tilapia Oreochromis niloticus (L.), blue tilapia, Oreochromis aureus (Steind.) and their hybrids in Lake Edku, Egypt. Egyptian Journal of Aquatic Biology and Fisheries 6: 121–142. https://doi.org/10.21608/ejabf.2002.1753.
68 Jiménez-Badillo, L. 2006. Age-growth models for tilapia Oreochromis aureus (Perciformes, Cichlidae) of the Infiernillo reservoir, Mexico and reproductive behaviour. Revista de Biología Tropical 54: 577–588.
69 Peña Messina, Emilio, Raul Tapia Varela, José Iván Velázquez Abunader, Alma Araceli Orbe Mendoza, and Javier Marcial de Jesús Ruiz Velazco Arce. 2010. Growth, mortality and reproduction of the blue tilapia Oreochromis aureus (Perciformes: Cichlidae) in the Aguamilpa Reservoir, Mexico. Revista de Biología Tropical 58: 1577–1586.
70 Rana, Krishen. 1988. Reproductive Biology and the Hatchery Rearing of Tilapia Eggs and Fry. In Recent Advances in Aquaculture, ed. James F. Muir and Ronald J. Roberts, 343–406. Dordrecht: Springer Netherlands.
71 Salin, Krishna R., and Amara Yakupitiyage. 2023. A manual for Nile tilapia seed production and grow-out aquaculture. Penang, Malaysia: WorldFish.
72 Soltan, M. A., A. Z. Hegazi, S. M. M. El-Laithy, and A. F. Fath El-Bab. 2007. Effect of spawning season, male and female body weight on reproductive performance of Nile tilapia, Oreochromis niloticus. Journal of the Egyptian Veterinary Medical Association 67: 97–108.
73 Fessehaye, Yonas, Zizy El-bialy, Mahmoud A. Rezk, Richard Crooijmans, Henk Bovenhuis, and Hans Komen. 2006. Mating systems and male reproductive success in Nile tilapia (Oreochromis niloticus) in breeding hapas: A microsatellite analysis. Aquaculture 256: 148–158. https://doi.org/10.1016/j.aquaculture.2006.02.024.
74 Fayed, W., A. El-Dahhar, S. El-Zaeem, Z. El-Greisy, M. Salama, and G. Sallam. 2014. Influence of Protein Levels and Pond Fertilization during Broodstock Pre-Spawning Period on the Tolerance of Nile Tilapia, Oreochromis niloticus, Fry to Winter Season Temperature. Mediterranean Aquaculture Journal 6: 1–13. https://doi.org/10.21608/maj.2014.4623.
75 Maia, C.M. 2024. Personal communication.
76 Vera Cruz, E. M., and G. C. Mair. 1994. Conditions for effective androgen sex reversal in Oreochromis niloticus (L.). Aquaculture 122: 237–248. https://doi.org/10.1016/0044-8486(94)90513-4.
77 Gilbert, P. 1996. Breeding and propagation of tilapia (Oreochromis niloticus) in a floating hatchery, Gabon. Naga, the ICLARM Quarterly 19: 26–33.
78 Abaho, Ivan, Thaddeus Zaabwe, Andrew Izaara, Howard N. Kasigwa, Norman Mushabe, Steven Byenkya, Mujibu Nkambo, Sylvester D. Baguma, David L. N. Hafashimana, and Jackson Efitre. 2020. Effect of stocking density on growth and survival of Nile tilapia (Oreochromis niloticus, Linnaeus 1758) under cage culture in Lake Albert, Uganda. International Journal of Fisheries and Aquaculture 12: 26–35. https://doi.org/10.5897/IJFA2018.0671.
79 Melard, C. 1995. Production of a high percentage of male offspring with 17α-ethynylestradiol sex-reversed Oreochromis aureus. I. Estrogen sex-reversal and production of F2 pseudofemales. Aquaculture 130: 25–34. https://doi.org/10.1016/0044-8486(94)00313-D.
80 Macintosh, D. J., and S. S. De Silva. 1984. The influence of stocking density and food ration on fry survival and growth in Oreochromis mossambicus and O. niloticus female × O. aureus male hybrids reared in a closed circulated system. Aquaculture 41: 345–358. https://doi.org/10.1016/0044-8486(84)90202-3.
81 Scoppettone, G. G., J. A. Salgado, and M. B. Nielsen. 2005. Blue tilapia (Oreochromis aureus) predation on fishes in the Muddy River system, Clark County, Nevada. Western North American Naturalist 65: 410–414.
82 Zengeya, Tsungai Alfred, Anthony J. Booth, Armanda D. S. Bastos, and Christian T. Chimimba. 2011. Trophic interrelationships between the exotic Nile tilapia, Oreochromis niloticus and indigenous tilapiine cichlids in a subtropical African river system (Limpopo River, South Africa). Environmental Biology of Fishes 92: 479–489. https://doi.org/10.1007/s10641-011-9865-4.
83 Chapman, Lauren J., Colin A. Chapman, and Mark Chandler. 1996. Wetland ecotones as refugia for endangered fishes. Biological Conservation 78: 263–270. https://doi.org/10.1016/S0006-3207(96)00030-4.
84 Chapman, Lauren J., Colin A. Chapman, Richard Ogutu-Ohwayo, Mark Chandler, Les Kaufman, and Amanda E. Keiter. 1996. Refugia for Endangered Fishes from an Introduced Predator in Lake Nabugabo, Uganda. Conservation Biology 10: 554–561. https://doi.org/10.1046/j.1523-1739.1996.10020554.x.
85 Rosenberger, A. E., and L. J. Chapman. 1999. Hypoxic wetland tributaries as faunal refugia from an introduced predator. Ecology of Freshwater Fish 8: 22–34. https://doi.org/10.1111/j.1600-0633.1999.tb00049.x.
86 Schofield, Pamela J., and Lauren J. Chapman. 1999. Interactions Between Nile Perch, Lates niloticus, and Other Fishes in Lake Nabugabo, Uganda. Environmental Biology of Fishes 55: 343–358. https://doi.org/10.1023/A:1007544017989.
87 Goren, M. 1974. The freshwater fishes of Israel. Israel Journal of Zoology 23: 67–118. https://doi.org/10.1080/00212210.1974.10688400.
88 El-Zaeem, S. Y. 2011. Growth comparison of Nile tilapia (Oreochromis niloticus) and Blue tilapia, (Oreochromis aureus) as affected by classical and modern breeding methods. African Journal of Biotechnology 10: 12071–12078.
89 Vera Cruz, Emmanuel M., Eddie Boy T. Jimenez, and Zaldy P. Bartolome. 2023. Shading Influenced Water Quality and Seed Production of Nile Tilapia (Oreochromis niloticus L.) in the Hapa-within-Pond System During Warm Months. The Philippine Journal of Fisheries 30: 155–161. https://doi.org/10.31398/tpjf/30.2.2022-0026.
90 Rana, Kausik J. 1986. Parental influences on egg quality, fry production and fry performance in Oreochromis niloticus (Linnaeus) and O. mossambicus (Peters). Doctoral dissertation, U.K.: University of Stirling.
91 Obirikorang, Kwasi Adu, Esther Adu Yeboah, Benjamin Apraku Gyampoh, and Seyram Kwadzo Amanie-Adjei. 2022. Effect of road conditions on physiological stress responses and post-transportation growth and survival of Nile tilapia (Oreochromis niloticus) fingerlings. Journal of Applied Aquaculture 34: 180–196. https://doi.org/10.1080/10454438.2020.1825269.
92 Saboya, J. P. S., G. S. Araujo, J. W. A. Silva, J. Sousa Junior, R. L. Maciel, and W. R. L. Farias. 2012. Efeito dos polissacarídeos sulfatados da rodofícea Kappaphycus alvarezii em pós-larvas de tilápia do Nilo (Oreochromis niloticus) submetidas a situações de estresse. Acta Scientiarum. Animal Sciences 34: 215–221. https://doi.org/10.4025/actascianimsci.v34i3.13213.
93 Barcellos, Leonardo José Gil, S. Nicolaiewsky, S M G. De Souza, and F. Lulhier. 1999. The effects of stocking density and social interaction on acute stress response in Nile tilapia Oreochromis niloticus (L.) fingerlings. Aquaculture Research 30: 887–892. https://doi.org/10.1046/j.1365-2109.1999.00419.x.
94 Barcellos, Leonardo José Gil, Gilson Luiz Volpato, Rodrigo Egydio Barreto, Ivanir Coldebella, and Daiane Ferreira. 2011. Chemical communication of handling stress in fish. Physiology & Behavior 103: 372–375. https://doi.org/10.1016/j.physbeh.2011.03.009.
95 Deriggi, Graziele Fernanda, Luis Antonio Kioshi Aoki Inoue, and Moraes Gilberto. 2006. Stress responses to handling in Nile tilapia (Oreochromis niloticus Linnaeus): assessment of eugenol as an alternative anesthetic = Respostas metabólicas da tilápia do Nilo (Oreochromis niloticus) submetida ao manuseio e ao anestésico eugenol. ResearchGate 28.
96 Simões, L. N., A. T. M. Gomide, V. M. F. Almeida-Val, A. L. Val, and L. C. Gomes. 2012. O uso do óleo de cravo como anestésico em juvenis avançados de tilápia do Nilo (Oreochromis niloticus). Acta Scientiarum. Animal Sciences 34: 175–181. https://doi.org/10.4025/actascianimsci.v34i2.13022.
97 Mustapha, Moshood Keke, Olatoyosi Taofeek Oladokun, Mubarak Mayowa Salman, Idris Adewale Adeniyi, and Dele Ojo. 2014. Does light duration (photoperiod) have an effect on the mortality and welfare of cultured Oreochromis niloticus and Clarias gariepinus? Turkish Journal of Zoology 38: 466–470.
98 Maia, Caroline Marques, and Gilson Luiz Volpato. 2013. Environmental light color affects the stress response of Nile tilapia. Zoology 116: 64–66. https://doi.org/10.1016/j.zool.2012.08.001.
99 Martins, Catarina I. M., Patricia I. M. Silva, Luis E. C. Conceição, Benjamin Costas, Erik Höglund, Øyvind Øverli, and Johan W. Schrama. 2011. Linking fearfulness and coping styles in fish. PLOS ONE 6: e28084. https://doi.org/10.1371/journal.pone.0028084.
100 El-Hawarry, Waleed N., Radi A. Mohamed, and Safinaz A. Ibrahim. 2018. Collaborating effects of rearing density and oregano oil supplementation on growth, behavioral and stress response of Nile tilapia (Oreochromis niloticus). Egyptian Journal of Aquatic Research 44: 173–178. https://doi.org/10.1016/j.ejar.2018.06.008.
101 Neto, João Favero, and Percilia Cardoso Giaquinto. 2020. Environmental enrichment techniques and tryptophan supplementation used to improve the quality of life and animal welfare of Nile tilapia. Aquaculture Reports 17: 100354. https://doi.org/10.1016/j.aqrep.2020.100354.
102 Saraiva, J. L., Margarida Nogueirinha, Rita Teodósio, Cláudia Aragão, Sofia Engrola, and Pablo Arechavala-Lopez. 2021. The effect of tank cover on welfare of farmed Nile tilapia. Applied Animal Behaviour Science: 105396. https://doi.org/10.1016/j.applanim.2021.105396.
103 Cnaani, Avner, Simon Tinman, Yaacov Avidar, Micha Ron, and Gideon Hulata. 2004. Comparative study of biochemical parameters in response to stress in Oreochromis aureus, O. mossambicus and two strains of O. niloticus. Aquaculture Research 35: 1434–1440. https://doi.org/10.1111/j.1365-2109.2004.01167.x.
104 Yang, Changgeng, Hua Fan, Liya Ge, Qian Ma, Ming Jiang, and Hua Wen. 2023. Comparative analysis of quantitative phosphoproteomics between two tilapias (Oreochromis niloticus and Oreochromis aureus) under low-temperature stress. PeerJ 11: e15599. https://doi.org/10.7717/peerj.15599.
105 Martins, Maurício Laterça, Daniela Takahashi Nomura, Danilo Makoto Yamaguchi Myiazaki, Fabiana Pilarsky, Karina Ribeiro, Marcello Pardi De Castro, and Cristiane Fátima Meldau De Campos. 2004. Physiological and haematological response of Oreochromis niloticus (Osteichthyes: Cichlidae) exposed to single and consecutive stress of capture. Acta Scientiarum. Animal Sciences 26: 449–456. https://doi.org/10.4025/actascianimsci.v26i4.1719.
106 Barreto, Rodrigo Egydio, and Gilson Luiz Volpato. 2007. Evaluating feeding as unconditioned stimulus for conditioning of an endocrine effect in Nile tilapia. Physiology & Behavior 92: 867–872. https://doi.org/10.1016/j.physbeh.2007.06.013.
107 Luchiari, A. C., and F. a. M. Freire. 2009. Effects of environmental colour on growth of Nile tilapia, Oreochromis niloticus (Linnaeus, 1758), maintained individually or in groups. Journal of Applied Ichthyology 25: 162–167. https://doi.org/10.1111/j.1439-0426.2008.01203.x.
108 Barreto, Rodrigo Egydio, and Gilson Luiz Volpato. 2004. Caution for using ventilatory frequency as an indicator of stress in fish. Behavioural Processes 66: 43–51. https://doi.org/10.1016/j.beproc.2004.01.001.
109 Volpato, G. L., and R. E. Barreto. 2001. Environmental blue light prevents stress in the fish Nile tilapia. Brazilian Journal of Medical and Biological Research 34: 1041–1045. https://doi.org/10.1590/S0100-879X2001000800011.
110 Smith, Michael E., Andrew S. Kane, and Arthur N. Popper. 2004. Acoustical stress and hearing sensitivity in fishes: does the linear threshold shift hypothesis hold water? Journal of Experimental Biology 207: 3591–3602. https://doi.org/10.1242/jeb.01188.
111 Roques, Jonathan A. C., Wout Abbink, Femke Geurds, Hans van de Vis, and Gert Flik. 2010. Tailfin clipping, a painful procedure: Studies on Nile tilapia and common carp. Physiology & Behavior 101: 533–540. https://doi.org/10.1016/j.physbeh.2010.08.001.
112 Barreto, Rodrigo Egydio, and Gilson Luiz Volpato. 2006. Stress responses of the fish Nile tilapia subjected to electroshock and social stressors. Brazilian Journal of Medical and Biological Research 39: 1605–1612. https://doi.org/10.1590/S0100-879X2006001200012.
113 Barreto, Rodrigo Egydio, and Gilson Luiz Volpato. 2006. Ventilatory frequency of Nile tilapia subjected to different stressors. Journal of Experimental Animal Science 43: 189–196. https://doi.org/10.1016/j.jeas.2006.05.001.
114 Akar, Adel M. A. 2011. Effects of Clove Oil on the Response of Blue Tilapia ( Oreochromis Aureus) by Transportation Stress. Journal of the Arabian Aquaculture Society 6: 77–86.
115 Fessehaye, Yonas, Hans Komen, Mahmoud A. Rezk, Johan A.M. van Arendonk, and Henk Bovenhuis. 2007. Effects of inbreeding on survival, body weight and fluctuating asymmetry (FA) in Nile tilapia, Oreochromis niloticus. Aquaculture 264: 27–35. https://doi.org/10.1016/j.aquaculture.2006.12.038.
116 Eissa, A.E., M. Moustafa, I.N. El-Husseiny, S. Saeid, O. Saleh, and T. Borhan. 2009. Identification of some skeletal deformities in freshwater teleosts raised in Egyptian aquaculture. Chemosphere 77: 419–425. https://doi.org/10.1016/j.chemosphere.2009.06.050.
117 The fish people. 2016. The Tilapia handbook 3.0. Western Edge Seafood.
118 Xu, Pao, and Junchao Ming. 2018. Status and Trends of the Tilapia Farming Industry Development. In Aquaculture in China: Success stories and modern trends, 404–420. John Wiley & Sons, Ltd.
119 Agyakwah, Seth K., Ruby Asmah, Emmanual T. D. Mensah, Catherine Ragasa, Sena Amewu, Nhuong Tran, Mathew Oyih, and Peter Ziddah. 2020. Farmer’s manual on small-scale tilapia pond farming in Ghana.
120 Coelho, Meg, As Pedrazzani, Mh Quintiliano, F Bolfe, and Cfm Molento. 2022. Fish slaughter practices in Brazilian aquaculture and their consequences for animal welfare. Animal Welfare 31: 187–192. https://doi.org/10.7120/09627286.31.2.003.
121 Sonu. 2022. Developing high-welfare stunning solutions with Ace Aquatech. Regal Springs.
122 Teletchea, Fabrice, and Pascal Fontaine. 2012. Levels of domestication in fish: implications for the sustainable future of aquaculture. Fish and Fisheries 15: 181–195. https://doi.org/10.1111/faf.12006.
123 Zambrano, Luis, Elsa Valiente, and M. Jake Vander Zanden. 2010. Food web overlap among native axolotl (Ambystoma mexicanum) and two exotic fishes: carp (Cyprinus carpio) and tilapia (Oreochromis niloticus) in Xochimilco, Mexico City. Biological Invasions 12: 3061–3069. https://doi.org/10.1007/s10530-010-9697-8.
124 Traxler, S. L., and B. Murphy. 1995. Experimental trophic ecology of juvenile largemouth bass,Micropterus salmoides, and blue tilapia,Oreochromis aureus. Environmental Biology of Fishes 42: 201–211. https://doi.org/10.1007/BF00001998.
125 Jiménez-Badillo, M. L., and M. R. Nepita-Villanueva. 2000. [Trophic range of tilapia Oreochromis aureus (Perciformes: Cichlidae) in Infiernillo dam, Michoacán-Guerrero, Mexico]. Revista De Biologia Tropical 48: 487–494.
126 Fall, J., Y.-T. Tseng, D. Ndong, and S.-S. Sheen. 2011. The effects of different protein sources on the growth of hybrid tilapia (Oreochromis niloticus × O. aureus) reared under fresh water and brackish water. African Journal of Agricultural Research 6: 5024–5029.
127 Coyle, S. D., G. J. Mengel, J. H. Tidwell, and C. D. Webster. 2004. Evaluation of growth, feed utilization, and economics of hybrid tilapia, Oreochromis niloticus×Oreochromis aureus, fed diets containing different protein sources in combination with distillers dried grains with solubles. Aquaculture Research 35: 365–370. https://doi.org/10.1111/j.1365-2109.2004.01023.x.
128 Shiau, S.-Y., S.-F. Lin, S.-L. Yu, A.-L. Lin, and C.-C. Kwok. 1990. Defatted and full-fat soybean meal as partial replacements for fishmeal in tilapia (Oreochromis niloticus×O. aureus) diets at low protein level. Aquaculture 86: 401–407. https://doi.org/10.1016/0044-8486(90)90328-K.
129 Lin, S., and L. Luo. 2011. Effects of different levels of soybean meal inclusion in replacement for fish meal on growth, digestive enzymes and transaminase activities in practical diets for juvenile tilapia, Oreochromis niloticus × O. aureus. Animal Feed Science and Technology 168: 80–87. https://doi.org/10.1016/j.anifeedsci.2011.03.012.
130 Shiau, S.-Y., J.-L. Chuang, and C.-L. Sun. 1987. Inclusion of soybean meal in tilapia (Oreochromis niloticus × O. aureus) diets at two protein levels. Aquaculture 65: 251–261. https://doi.org/10.1016/0044-8486(87)90238-9.
131 Qiao, Y., K. Mai, and Q. Ai. 2019. Effects of Fish Meal Replaced by Maggot Culture on Growth Performance, Body Composition, and Antioxidant Responses of Hybrid Tilapia (Oreochromis niloticus × O. aureus). The Israeli Journal of Aquaculture 71: 1606.
132 Han, C. -Y., Q. -M. Zheng, and L. -N. Feng. 2013. Effects of total replacement of dietary fish oil on growth performance and fatty acid compositions of hybrid tilapia (Oreochromis niloticus × O. aureus). Aquaculture International 21: 1209–1217. https://doi.org/10.1007/s10499-013-9624-y.
133 Deng, J., K. Wang, K. Mai, L. Chen, L. Zhang, and H. Mi. 2017. Effects of replacing fish meal with rubber seed meal on growth, nutrient utilization, and cholesterol metabolism of tilapia (Oreochromis niloticus × O. aureus). Fish Physiology and Biochemistry 43: 941–954. https://doi.org/10.1007/s10695-016-0313-4.
134 Mood, Alison, Elena Lara, Natasha K. Boyland, and Phil Brooke. 2023. Estimating global numbers of farmed fishes killed for food annually from 1990 to 2019. Animal Welfare 32: e12. https://doi.org/10.1017/awf.2023.4.
Lorem ipsum
Something along the lines of: we were aware of the importance of some topics so that we wanted to include them and collect data but not score them. For WelfareChecks | farm, these topics are "domestication level", "feed replacement", and "commercial relevance". The domestication and commercial relevance aspects allow us to analyse the questions whether increasing rate of domestication or relevance in farming worldwide goes hand in hand with better welfare; the feed replacement rather goes in the direction of added suffering for all those species which end up as feed. For a carnivorous species, to gain 1 kg of meat, you do not just kill this one individual but you have to take into account the meat that it was fed during its life in the form of fish meal and fish oil. In other words, carnivorous species (and to a degree also omnivorous ones) have a larger "fish in:fish out" ratio.
Lorem ipsum
Probably, we updated the profile. Check the version number in the head of the page. For more information on the version, see the FAQ about this. Why do we update profiles? Not just do we want to include new research that has come out, but we are continuously developing the database itself. For example, we changed the structure of entries in criteria or we added explanations for scores in the WelfareCheck | farm. And we are always refining our scoring rules.
The centre of the Overview is an array of criteria covering basic features and behaviours of the species. Each of this information comes from our literature search on the species. If we researched a full Dossier on the species, probably all criteria in the Overview will be covered and thus filled. This was our way to go when we first set up the database.
Because Dossiers are time consuming to research, we switched to focusing on WelfareChecks. These are much shorter profiles covering just 10 criteria we deemed important when it comes to behaviour and welfare in aquaculture (and lately fisheries, too). Also, WelfareChecks contain the assessment of the welfare potential of a species which has become the main feature of the fair-fish database over time. Because WelfareChecks do not cover as many criteria as a Dossier, we don't have the information to fill all blanks in the Overview, as this information is "not investigated by us yet".
Our long-term goal is to go back to researching Dossiers for all species covered in the fair-fish database once we set up WelfareChecks for each of them. If you would like to support us financially with this, please get in touch at ffdb@fair-fish.net
See the question "What does "not investigated by us yet" mean?". In short, if we have not had a look in the literature - or in other words, if we have not investigated a criterion - we cannot know the data. If we have already checked the literature on a criterion and could not find anything, it is "no data found yet". You spotted a "no data found yet" where you know data exists? Get in touch with us at ffdb@fair-fish.net!
Once you have clicked on "show details", the entry for a criterion will unfold and display the summarised information we collected from the scientific literature – complete with the reference(s).
As reference style we chose "Springer Humanities (numeric, brackets)" which presents itself in the database as a number in a grey box. Mouse over the box to see the reference; click on it to jump to the bibliography at the bottom of the page. But what does "[x]-[y]" refer to?
This is the way we mark secondary citations. In this case, we read reference "y", but not reference "x", and cite "x" as mentioned in "y". We try to avoid citing secondary references as best as possible and instead read the original source ourselves. Sometimes we have to resort to citing secondarily, though, when the original source is: a) very old or not (digitally) available for other reasons, b) in a language no one in the team understands. Seldomly, it also happens that we are running out of time on a profile and cannot afford to read the original. As mentioned, though, we try to avoid it, as citing mistakes may always happen (and we don't want to copy the mistake) and as misunderstandings may occur by interpreting the secondarily cited information incorrectly.
If you spot a secondary reference and would like to send us the original work, please contact us at ffdb@fair-fish.net
In general, we aim at giving a good representation of the literature published on the respective species and read as much as we can. We do have a time budget on each profile, though. This is around 80-100 hours for a WelfareCheck and around 300 hours for a Dossier. It might thus be that we simply did not come around to reading the paper.
It is also possible, though, that we did have to make a decision between several papers on the same topic. If there are too many papers on one issue than we manage to read in time, we have to select a sample. On certain topics that currently attract a lot of attention, it might be beneficial to opt for the more recent papers; on other topics, especially in basic research on behaviour in the wild, the older papers might be the go-to source.
And speaking of time: the paper you are missing from the profile might have come out after the profile was published. For the publication date, please check the head of the profile at "cite this profile". We currently update profiles every 6-7 years.
If your paper slipped through the cracks and you would like us to consider it, please get in touch at ffdb@fair-fish.net
This number, for example "C | 2.1 (2022-11-02)", contains 4 parts:
- "C" marks the appearance – the design level – of the profile part. In WelfareChecks | farm, appearance "C" is our most recent one with consistent age class and label (WILD, FARM, LAB) structure across all criteria.
- "2." marks the number of major releases within this appearance. Here, it is major release 2. Major releases include e.g. changes of the WelfareScore. Even if we just add one paper – if it changes the score for one or several criteria, we will mark this as a major update for the profile. With a change to a new appearance, the major release will be re-set to 1.
- ".1" marks the number of minor updates within this appearance. Here, it is minor update 1. With minor updates, we mean changes in formatting, grammar, orthography. It can also mean adding new papers, but if these papers only confirm the score and don't change it, it will be "minor" in our book. With a change to a new appearance, the minor update will be re-set to 0.
- "(2022-11-02)" is the date of the last change – be it the initial release of the part, a minor, or a major update. The nature of the changes you may find out in the changelog next to the version number.
If an Advice, for example, has an initial release date and then just a minor update date due to link corrections, it means that – apart from correcting links – the Advice has not been updated in a major way since its initial release. Please take this into account when consulting any part of the database.
Lorem ipsum
In the fair-fish database, when you have chosen a species (either by searching in the search bar or in the species tree), the landing page is an Overview, introducing the most important information to know about the species that we have come across during our literatures search, including common names, images, distribution, habitat and growth characteristics, swimming aspects, reproduction, social behaviour but also handling details. To dive deeper, visit the Dossier where we collect all available ethological findings (and more) on the most important aspects during the life course, both biologically and concerning the habitat. In contrast to the Overview, we present the findings in more detail citing the scientific references.
Depending on whether the species is farmed or wild caught, you will be interested in different branches of the database.
Farm branch
Founded in 2013, the farm branch of the fair-fish database focuses on farmed aquatic species.
Catch branch
Founded in 2022, the catch branch of the fair-fish database focuses on wild-caught aquatic species.
The heart of the farm branch of the fair-fish database is the welfare assessment – or WelfareCheck | farm – resulting in the WelfareScore | farm for each species. The WelfareCheck | farm is a condensed assessment of the species' likelihood and potential for good welfare in aquaculture, based on welfare-related findings for 10 crucial criteria (home range, depth range, migration, reproduction, aggregation, aggression, substrate, stress, malformations, slaughter).
For those species with a Dossier, we conclude to-be-preferred farming conditions in the Advice | farm. They are not meant to be as detailed as a rearing manual but instead, challenge current farming standards and often take the form of what not to do.
In parallel to farm, the main element of the catch branch of the fair-fish database is the welfare assessment – or WelfareCheck | catch – with the WelfareScore | catch for each species caught with a specific catching method. The WelfareCheck | catch, too, is a condensed assessment of the species' likelihood and potential for good welfare – or better yet avoidance of decrease of good welfare – this time in fisheries. We base this on findings on welfare hazards in 10 steps along the catching process (prospection, setting, catching, emersion, release from gear, bycatch avoidance, sorting, discarding, storing, slaughter).
In contrast to the farm profiles, in the catch branch we assess the welfare separately for each method that the focus species is caught with. In the case of a species exclusively caught with one method, there will be one WelfareCheck, whereas in other species, there will be as many WelfareChecks as there are methods to catch the species with.
Summarising our findings of all WelfareChecks | catch for one species in Advice | catch, we conclude which catching method is the least welfare threatening for this species and which changes to the gear or the catching process will potentially result in improvements of welfare.
Try mousing over the element you are interested in - oftentimes you will find explanations this way. If not, there will be FAQ on many of the sub-pages with answers to questions that apply to the respective sub-page. If your question is not among those, contact us at ffdb@fair-fish.net.
It's right here! We decided to re-name it to fair-fish database for several reasons. The database has grown beyond dealing purely with ethology, more towards welfare in general – and so much more. Also, the partners fair-fish and FishEthoGroup decided to re-organise their partnership. While maintaining our friendship, we also desire for greater independence. So, the name "fair-fish database" establishes it as a fair-fish endeavour.